If you are looking for a way to convert secondary alcohols to ketones without affecting other sensitive functional groups, you might want to consider the Oppenauer oxidation. This is a classic organic reaction that was discovered by Rupert Viktor Oppenauer in 1912. In this blog post, I will explain what the Oppenauer oxidation is, how it works, and what are some of its advantages and disadvantages.
The Oppenauer oxidation is a method for selectively oxidizing secondary alcohols to ketones using aluminium alkoxides [Al(OBut)3 and Al(OPri)3 ] as catalysts and carbonyl compounds (eg. Acetone) as hydride acceptors. It is a classical yet useful method and is widely employed for the synthesis of steroids and terpenoids. The oxidation is highly selective for secondary alcohols and does not oxidize other sensitive functional groups such as amines, and sulfides. Though primary alcohols can be oxidized under Oppenauer conditions, primary alcohols are seldom oxidized by this method due to the competing aldol condensation of aldehyde products. The reaction is the opposite of the Meerwein-Ponndorf-Verley reduction, which reduces ketones to secondary alcohols using the same reagents.

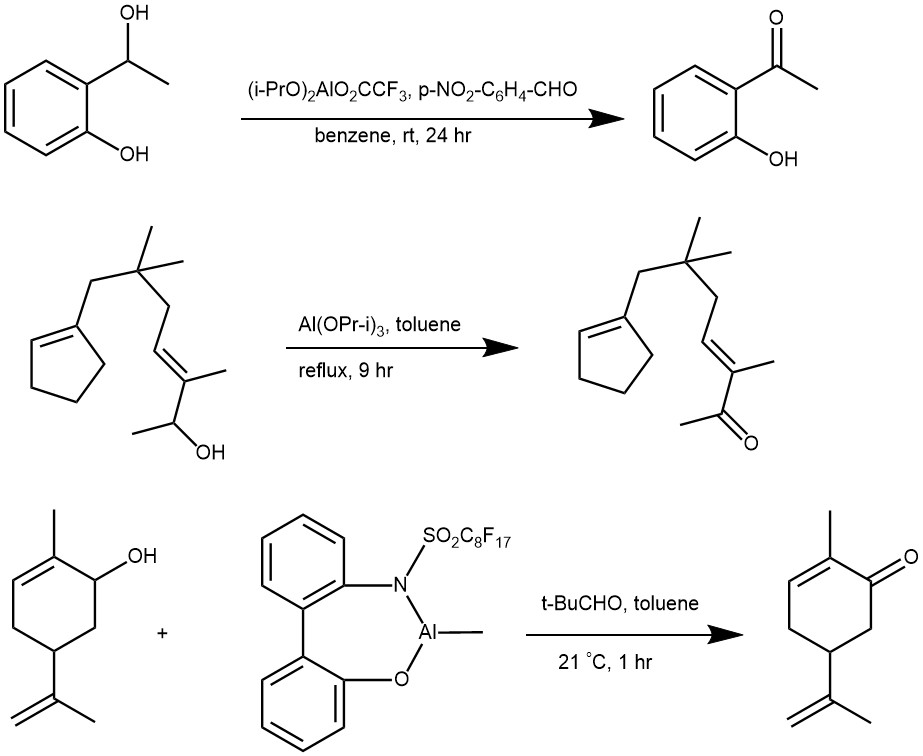
MECHANISM: Oppenauer oxidation proceeds through a process known as hydride transfer. The reaction involves the use of an aluminum alkoxide catalyst, such as aluminum isopropoxide, and a carbonyl compound, such as acetone, benzophenone, fluorenone, etc. as a hydrogen acceptor. The reaction is typically carried out in an organic solvent, such as dichloromethane or ethanol.
In the first step of the reaction, the aluminum alkoxide catalyst reacts with the alcohol to form an alkoxide intermediate. The carbonyl compound (acetone) then coordinates with the alkoxide intermediate to form alcohol/acetone aluminum complex. The hydride transfer occurs via a six-membered transition state followed by hydrolysis to regenerate the aluminum alkoxide catalyst.
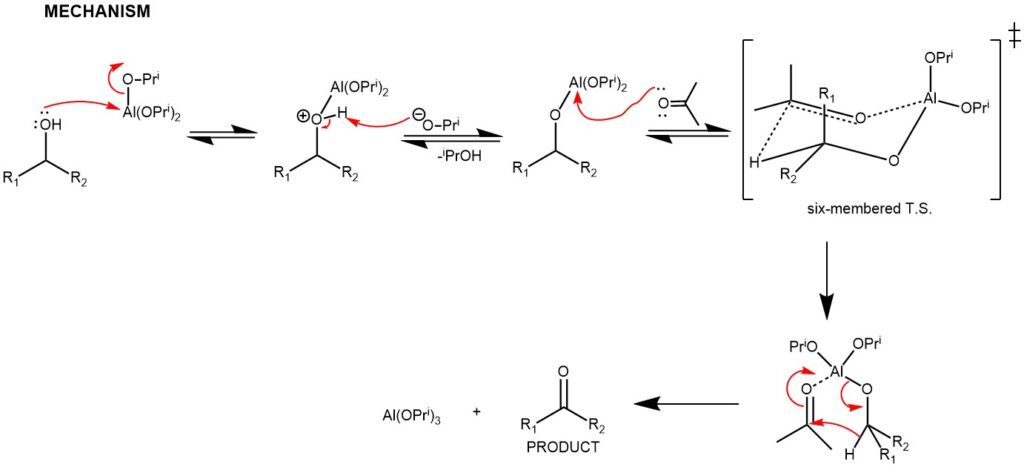
EXAMPLE 1: 2-Ethoxyestra-1,3,5(10)-trien-3,17-diol (1.88 g, 5.67 mmol) was placed in a 250 mL round bottom flask that was equipped with a 25 mL Dean-Stark trap and a reflux condenser. The entire apparatus had been flame dried under an argon atmosphere. Toluene (50 mL) was added to dissolve the Starting material. Aluminum isopropoxide (5.7g, 28.4 mmol) and cyclohexanone (23.5 mL, 2.26.8 mmol) were added and the entire reaction mixture was heated at reflux (145-150° C.) for 20 h. Saturated aqueous Sodium bicarbonate Solution (100 mL) was added after the reaction mixture was allowed to cool to room temperature. The organic material was extracted with dichloromethane (3×150 mL). The aqueous emulsion was acidified with 3 N HCl (~20 mL) until the emulsion separated and again, the aqueous layer was extracted with ethyl acetate (2×75 mL). The combined organic extracts were dried over magnesium Sulfate and condensed in vacuo. The crude product was purified via flash chromatography (silica gel, hexanes ethyl acetate 8:1 by Volume) to produce as a white solid (1.7g, 94%). [REF: US 2005/0014737 A1]
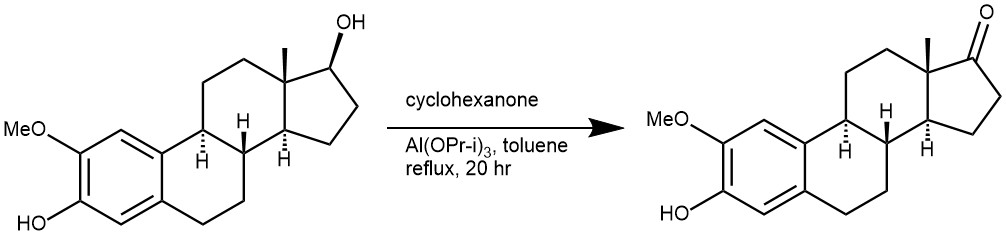
EXAMPLE 2: A mixture of cyclopamine 1 (11.29 g, 27.4 mmol, 1 equiv.), aluminum isopropoxide (5.60 g, 27.4 mmol, 1 equiv.), and 113 mL 2-butanone was heated at
reflux for 6.5 h. The mixture was cooled and 700 mL EtOAc and 700 ml Rochelle’s salt solutions were added and stirred for 2 h. The aqueous layer was diluted with EtOAc and 1 M NaOH, the organic layers were collected, dried over Na2SO4, filtered, and
concentrated under reduced pressure. The residue was purified by silica gel
chromatography on a Biotage™ system using CH2Cl2-EtOAc:MeOH as eluent to give
3 as a white solid (9.57 g, 85%). [REF: J. Med. Chem. 2008, 51, 6646–6649]
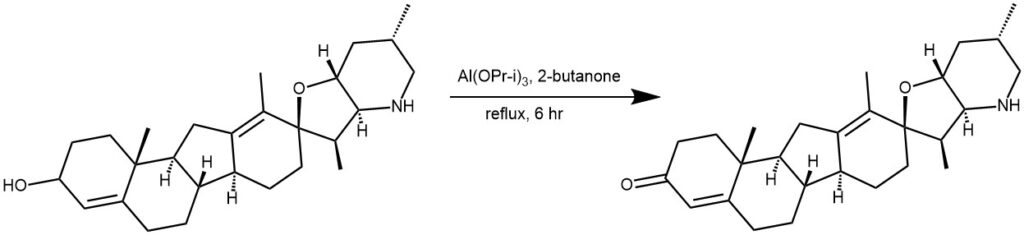
EXAMPLE 3: A mixture of potassium t-butoxide prepared from 0.5 g of potassium, 4.50 g of dry fluorenone, 2.02 g of dry cholesterol, and 40 mL of dry benzene was put under nitrogen and stirred with a magnetic stirrer for 1 hour at room temperature. The reaction mixture was diluted with water and ether. The ether layer was separated, dried and evaporated to leave 6.35 g of yellow oil. Crystallization from cyclohexane removed 2.99 g of fluorenone in three crops. The filtrate was then chromatographed on 100 g of alumina. It was finally purified by crystallization from cyclohexane to give 0.90 g product. [REF: J. Org. Chem. 1963, 28, 5, 1431–1433]
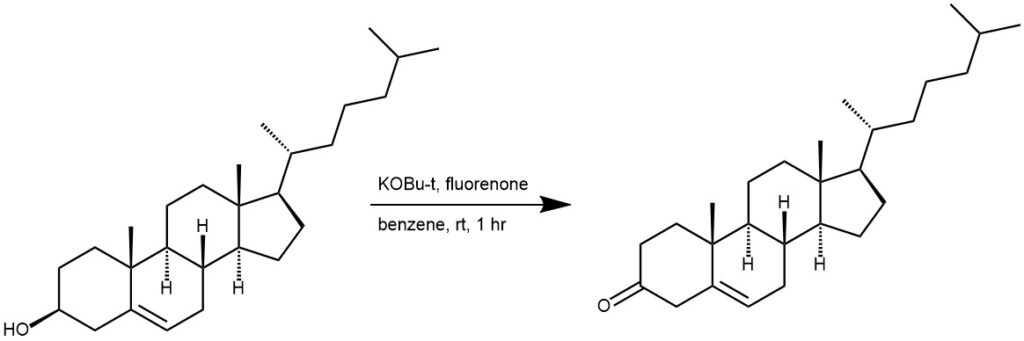
EXAMPLE 4: A stirred solution of 0.24 g of 2-adamantanol (1f) (1.6 mmol) in 10 mL of dichloromethane was treated with 0.1 mL of a 1.6 M solution of diethylethoxyaluminum in toluene (0.1 equiv) at room temperature. After 5 min, 0.3 mL of 1,1,1-trifluoroacetone (3.2 mmol)was added at once. Commercial 1,1,1-trifluoroacetone was used without further purification. The flask was tightly closed and allowed to stand at room temperature for 1 h. The reaction mixture was treated for 15 min with 0.25 mL of water and then with MgSO4. The solution was filtered and the solvent was removed under vacuum to yield 0.24 g of 2-adamantanone (2f) as a white solid. [REF: J. Org. Chem. 2007, 72, 9376-9378]

One of the main disadvantages of the Oppenauer oxidation is its low efficiency and atom economy. The reaction requires a large excess of both the aluminium alkoxide catalyst and the carbonyl compound hydride acceptor to drive the equilibrium towards the product side. This results in a lot of waste and byproducts that need to be separated and purified.
Another disadvantage of the Oppenauer oxidation is its limited scope and applicability. The reaction only works well for secondary alcohols that can form stable six-membered transition states with the aluminium catalyst. Primary alcohols, tertiary alcohols, phenols, and hindered alcohols are either unreactive or give poor yields under Oppenauer conditions. Furthermore, some carbonyl compounds such as esters, amides, lactones, and lactams are not suitable as hydride acceptors because they can undergo side reactions with the alcohol or alkoxide intermediates.The Oppenauer oxidation has also been largely displaced by newer and more efficient oxidation methods based on chromates (e.g., pyridinium chlorochromate), dimethyl sulfoxide (e.g., Swern oxidation), or hypervalent iodine (e.g IBX, DMP)
REFERENCES:
- Strategic applications of named reactions in organic synthesis by Laszlo Kurti, Barbara Czako
- Modern synthetic reactions by Herbert O. House